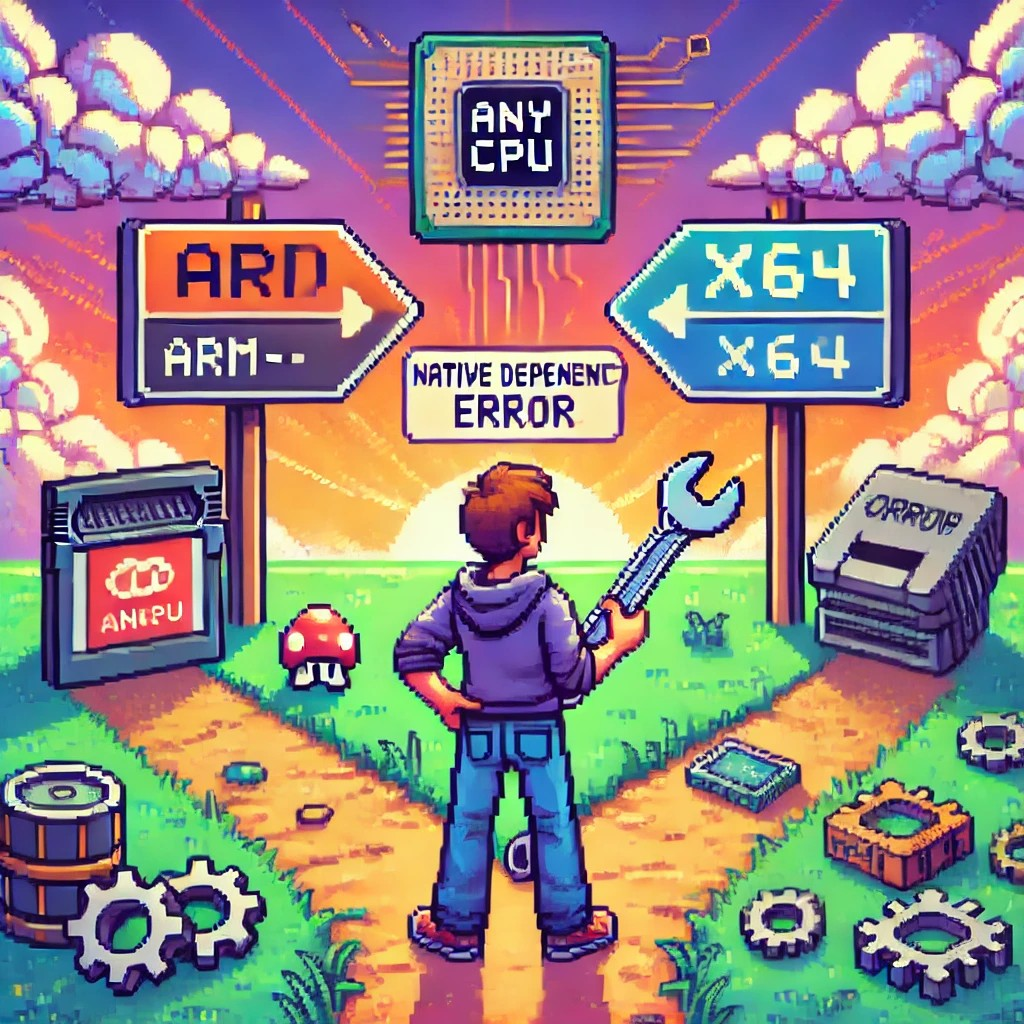
The AnyCPU Illusion: Native Dependencies in .NET Applications
Introduction
In the .NET ecosystem, “AnyCPU” is often considered a silver bullet for cross-platform deployment. However, this assumption can lead to significant problems when your application depends on native assemblies. In this post, I want to share a personal story that highlights how I discovered these limitations and how native dependencies affect the true portability of AnyCPU applications, especially for database access through ADO.NET and popular ORMs.
My Journey to Understanding AnyCPU’s Limitations
Every year, around Thanksgiving or Christmas, I visit my friend, brother, and business partner Javier. Two years ago, during one of these visits, I made a decision that would lead me to a pivotal realization about AnyCPU architecture.
At the time, I was tired of traveling with my bulky MSI GE72 Apache Pro-24 gaming laptop. According to MSI’s official specifications, it weighed 5.95 pounds—but that number didn’t include the hefty charger, which brought the total to around 12 pounds. Later, I upgraded to an MSI GF63 Thin, which was lighter at 4.10 pounds—but with the charger, it was still around 7.5 pounds. Lugging these laptops through airports felt like a workout.
Determined to travel lighter, I purchased a MacBook Air with the M2 chip. At just 2.7 pounds, including the charger, the MacBook Air felt like a breath of fresh air. The Apple Silicon chip was incredibly fast, and I immediately fell in love with the machine.
Having used a MacBook Pro with Bootcamp and Windows 7 years ago, I thought I could recreate that experience by running a Windows virtual machine on my MacBook Air to check projects and do some light development while traveling.
The Virtualization Experiment
As someone who loves virtualization, I eagerly set up a Windows virtual machine on my MacBook Air. I grabbed my trusty Windows x64 ISO, set up the virtual machine, and attempted to boot it—but it failed. I quickly realized the issue was related to CPU architecture. My x64 ISO wasn’t compatible with the ARM-based M2 chip.
Undeterred, I downloaded a Windows 11 ISO for ARM architecture and created the VM. Success! Windows was up and running, and I installed Visual Studio along with my essential development tools, including DevExpress XPO (my favorite ORM).
The Demo Disaster
The real test came during a trip to Dubai, where I was scheduled to give a live demo showcasing how quickly you can develop Line-of-Business (LOB) apps with XAF. Everything started smoothly until I tried to connect my XAF app to the database. Despite my best efforts, the connection failed.
In the middle of the demo, I switched to an in-memory data provider to salvage the presentation. After the demo, I dug into the issue and realized the root cause was related to the CPU architecture. The native database drivers I was using weren’t compatible with the ARM architecture.
A Familiar Problem
This situation reminded me of the transition from x86 to x64 years ago. Back then, I encountered similar issues where native drivers wouldn’t load unless they matched the process architecture.
The Native Dependency Challenge
Platform-Specific Loading Requirements
Native DLLs must exactly match the CPU architecture of your application:
- If your app runs as x86, it can only load x86 native DLLs.
- If running as x64, it requires x64 native DLLs.
- ARM requires ARM-specific binaries.
- ARM64 requires ARM64-specific binaries.
There is no flexibility—attempting to load a DLL compiled for a different architecture results in an immediate failure.
How Native Libraries are Loaded
When your application loads a native DLL, the operating system follows a specific search pattern:
- The application’s directory
- System directories (System32/SysWOW64)
- Directories listed in the PATH environment variable
Crucially, these native libraries must match the exact architecture of the running process.
// This seemingly simple code
[DllImport("native.dll")]
static extern void NativeMethod();
// Actually requires:
// - native.dll compiled for x86 when running as 32-bit
// - native.dll compiled for x64 when running as 64-bit
// - native.dll compiled for ARM64 when running on ARM64
The SQL Server Example
Let’s look at SQL Server connectivity, a common scenario where the AnyCPU illusion breaks down:
// Traditional ADO.NET connection
using (var connection = new SqlConnection(connectionString))
{
// This requires SQL Native Client
// Which must match the process architecture
await connection.OpenAsync();
}
Even though your application is compiled as AnyCPU, the SQL Native Client must match the process architecture. This becomes particularly problematic on newer architectures like ARM64, where native drivers may not be available.
Impact on ORMs
Entity Framework Core
Entity Framework Core, despite its modern design, still relies on database providers that may have native dependencies:
public class MyDbContext : DbContext
{
protected override void OnConfiguring(DbContextOptionsBuilder optionsBuilder)
{
// This configuration depends on:
// 1. SQL Native Client
// 2. Microsoft.Data.SqlClient native components
optionsBuilder.UseSqlServer(connectionString);
}
}
DevExpress XPO
DevExpress XPO faces similar challenges:
// XPO configuration
string connectionString = MSSqlConnectionProvider.GetConnectionString("server", "database");
XpoDefault.DataLayer = XpoDefault.GetDataLayer(connectionString, AutoCreateOption.DatabaseAndSchema);
// The MSSqlConnectionProvider relies on the same native SQL Server components
Solutions and Best Practices
1. Architecture-Specific Deployment
Instead of relying on AnyCPU, consider creating architecture-specific builds:
<PropertyGroup>
<Platforms>x86;x64;arm64</Platforms>
<RuntimeIdentifiers>win-x86;win-x64;win-arm64</RuntimeIdentifiers>
</PropertyGroup>
2. Runtime Provider Selection
Implement smart provider selection based on the current architecture:
public static class DatabaseProviderFactory
{
public static IDbConnection GetProvider()
{
return RuntimeInformation.ProcessArchitecture switch
{
Architecture.X86 => new SqlConnection(), // x86 native provider
Architecture.X64 => new SqlConnection(), // x64 native provider
Architecture.Arm64 => new Microsoft.Data.SqlClient.SqlConnection(), // ARM64 support
_ => throw new PlatformNotSupportedException()
};
}
}
3. Managed Fallbacks
Implement fallback strategies when native providers aren’t available:
public class DatabaseConnection
{
public async Task<IDbConnection> CreateConnectionAsync()
{
try
{
var connection = new SqlConnection(_connectionString);
await connection.OpenAsync();
return connection;
}
catch (DllNotFoundException)
{
var managedConnection = new Microsoft.Data.SqlClient.SqlConnection(_connectionString);
await managedConnection.OpenAsync();
return managedConnection;
}
}
}
4. Deployment Considerations
- Include all necessary native dependencies for each target architecture.
- Use architecture-specific directories in your deployment.
- Consider self-contained deployment to include the correct runtime.
Real-World Implications
This experience taught me that while AnyCPU provides excellent flexibility for managed code, it has limitations when dealing with native dependencies. These limitations become more apparent in scenarios like cloud deployments, ARM64 devices, and live demos.
Conclusion
The transition to ARM architecture is accelerating, and understanding the nuances of AnyCPU and native dependencies is more important than ever. By planning for architecture-specific deployments and implementing fallback strategies, you can build more resilient applications that can thrive in a multi-architecture world.